Pioneering DNA research aims to solve the mystery of cancer
Could a curveball called 'the blob' become a crucial weapon in the fight against incurable cancer and other diseases? Yes, in the hands of molecular biologist and biochemist Mats Nilsson it can. He is the mastermind behind ground-breaking technology turning Stockholm into an international hub for the mapping of our cells.
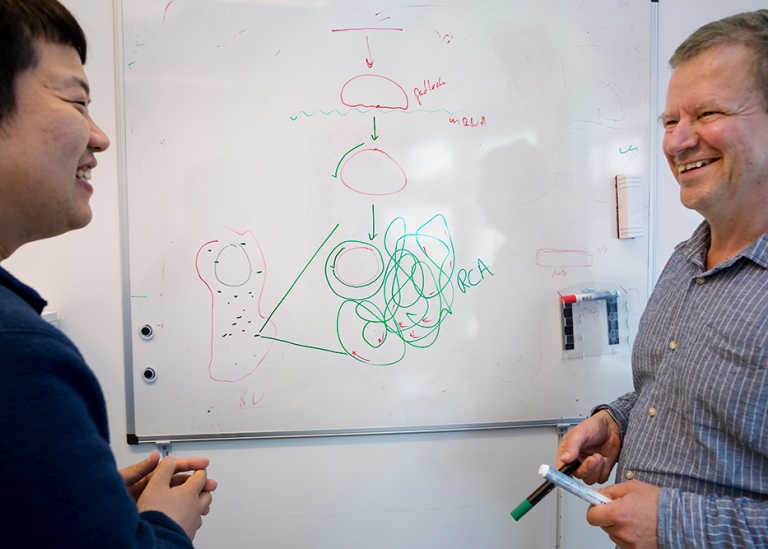
Throughout my research career, I have worked to make the molecules that build up life and our organs visible in different ways, says Mats Nilsson.
At the heart of his discovery is a so-called padlock probe, a small synthetic DNA molecule, which allows the DNA molecule to fluoresce in different colours, making it digitally visible in the tissue it is in. But to understand how groundbreaking it is, you first need to understand the basics.
How DNA, RNA and proteins work
Most organisms on Earth are single-celled. We humans, however, have a trillion cells in our bodies and are therefore called multicellular. These cells all carry a single molecule called DNA, our genetic material. Everything is written in the DNA code. How the cells should be built, how they should end up in the right place in the body and what their function should be.
"I think it's magic. Look at the arms, says Mats Nilsson, holding them up. They are quite similar, aren't they? How does the DNA code know that a cell should sit here and not there."
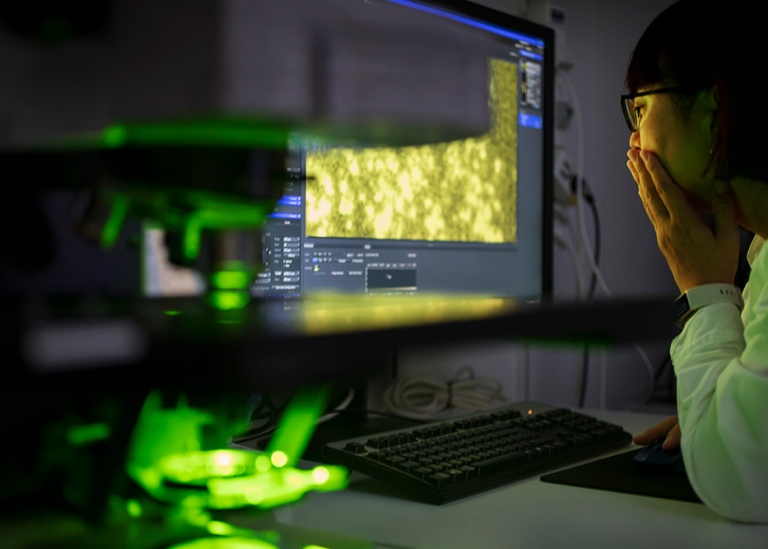
The DNA code as a cookbook
There are thousands of cell types in the body that are completely different from each other, even though they all carry the same DNA. For example, a skin cell is completely different from a nerve cell in the brain, or in the spine. Vision cells are sensitive to light and hearing cells sense vibrations in liquid. Each cell contains a set of molecules that carry information about what controls the active function of that particular cell. According to Mats Nilsson, the DNA code is like a cookbook written in just four letters, but with a total of three billion characters spread over 20 000 chapters. The recipes in the cookbook are our genes.
Each cell contains the entire scripture that tells us how we are constructed. The conditions for our imagination, everything we do and feel and experience are written here. Unlike the experiences we have in life, which are written in a different code in the brain, says Mats Nilsson.
RNA and proteins
The recipes, called RNA, can then be printed. RNA is a copy of DNA, but while DNA is in the cell nucleus, RNA is sent out of the nucleus and becomes more accessible. We will come back to that. But for there to be any 'action', another component is required, the proteins.
Proteins are the material that builds the cells and can also be sent out of a cell. In that case, they make contact with each other in a gap. In other words, cells are part of a protein network. Proteins are the component that can do things.
"They are like small machines. Some build up and others break down. Both functions are equally important. For example, they break down rubbish that enters the body. Other proteins are used to build. Occasionally there are errors such as plaque that causes Alzheimer's disease, or in relatively rare cases, a cancerous tumour. All the work is done by proteins."
The Blob - pioneering wetwear technology
WHAT IS A PADLOCK PROBE?
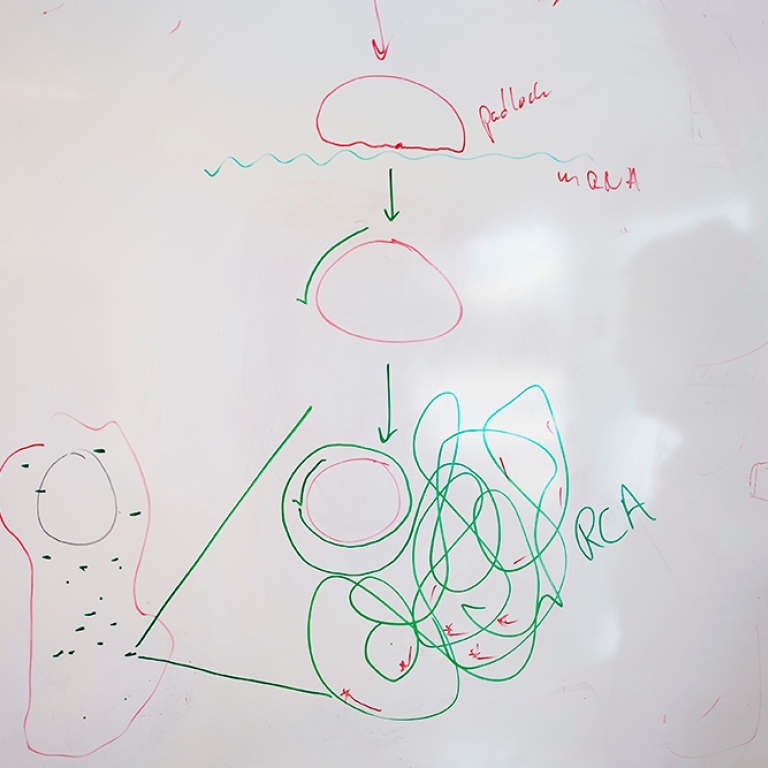
A padlock or a padlock probe is a DNA tool in the form of a piece of synthetic DNA, formed into a circle by an enzyme and specially designed to recognise a particular target molecule.
Once the circle is complete, another enzyme can then make a copy of the circle over and over again in an endless reaction into an ever-longer DNA strand with hundreds of copies of the DNA in the circle. The long strand of DNA eventually forms a nucleus, which has the ability to glow in a tissue sample using a simple fluorescence microscope. This allows different types of cells to become visible in a tissue sample. Using different colours, different cells can also be distinguished from each other in the tissue. For example, you can clearly see cancer cells in a tumour.
This is what I have been doing for thirty years out of sheer curiosity, and in the hope that it might be useful. I've been driven by the idea: Can you do this? Does this work? What could it be used for? Basically, I was hoping that it would be possible to visualise cancer cells and their mutations. Which it is.
In the meantime, development has moved at record speed. In 1998, the genome was not yet fully mapped and there were no sequencing machines. Genes were known, but not their DNA sequences. The chemistry available to map genes was slow and cumbersome.
"Back then, it took ten years to sequence a genome. Now it takes maybe 24 hours."
A 'eureka moment'
Mats’ invention is called 'nanoballs' or sometimes just 'blobs'. During a post-doc in Holland at a microscopy lab, he tried to go further with the crumbler to see if the fluorescent (amplifying) function worked.
"I looked in a microscope but saw nothing. It turned out that I had put in far too many. When I diluted the solution, I suddenly saw lots of them shining brightly."
It was one of many 'eureka moments' in his career. In total, there have been about five so far.
Research in the fight against cancer
HOW CANCER OCCURS
Cancer occurs due to damage in our DNA that changes the order of the code. It can lose a letter or whole blocks of letters, change the order, or add letters.
"There are many ways that errors can occur in DNA. Some changes allow cells to simply divide. As a trained biologist, I have Darwin's ideas deeply rooted in me and see it as a necessary consequence of natural selection, but here within an individual and not within a population of individuals."
However, it is not good for the body if cells start to lose discipline and divide spontaneously. They take over and form their own 'organs'.
We all have small mutations that live in our bodies. They don't kill us and it is actually bad luck if you get cancer. But then there are some that go off and become malignant. The next eureka moment for me would be if you can link what we know now to direct treatment, for example: killing all the green cells. Being able to help people who are sick. That's the next step.
Rapid technological developments in life sciences mean that the research team can now quickly and accurately, with the use of their own invention, the wetwear technology known as 'nanoballs' or 'blobs', distinguish between normal cells and cancer cells, as well as between different types of cancer cells. Clones. New mutations. New characteristics.
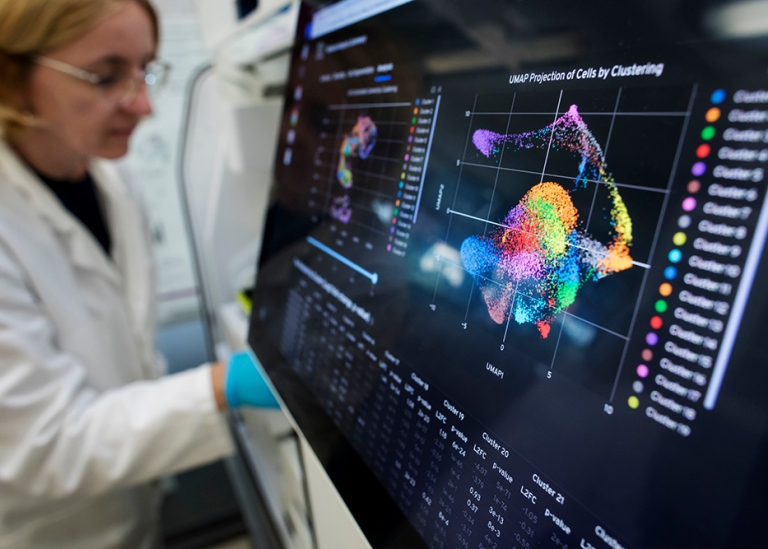
Can cancer become like any other disease?
"Yes, I think we will be able to cure cancer for a lot of people and keep it under control for others, like HIV. But we still haven't solved the complexity of cancer. There are so many different players that build up a tumour. Therefore, there is still a lot to be done. When we come up with new treatments that work, we don't always know why they work. It works for some but not for others. We try to understand why. That's the kind of information I think we can get with the new technology available now."
Are you there now? Or what is the next step?
I would like to do the mapping of DNA sequences in the same way that we have already sequenced RNA. And within more areas. To see the molecules of DNA in the nucleus of the cell itself glow. The question is: Can the same thing be done with DNA? If so, it would be very useful for the diagnosis and treatment of cancer. Most cancer mutations are not in RNA but in DNA. The difficulty here is that DNA is very compact.
And here we are back where Mats Nilsson started with the basic research question. Can it be done?
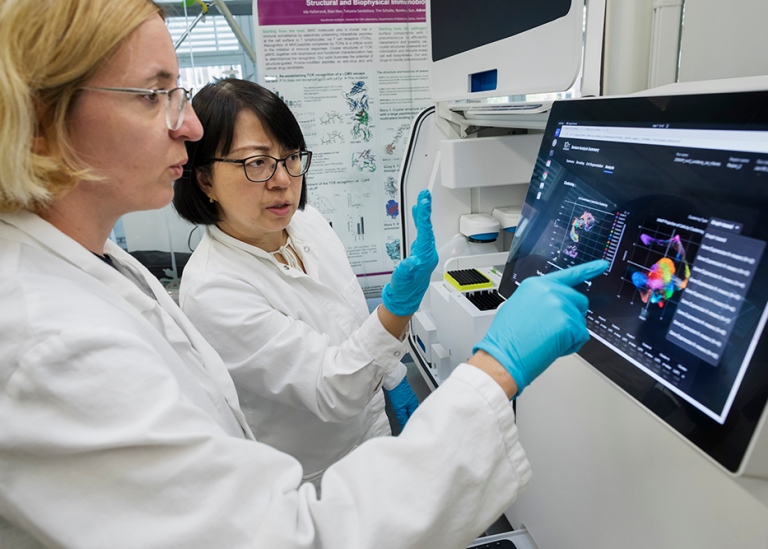
Rapid technology development in life sciences
KEY TECHNOLOGICAL ADVANCES
- 2000 - RNA, the code that DNA sends out as a recipe for activity in the body, has been possible to identify/map for the last 25 years.
- In 2008, it became possible to map DNA/RNA through efficient sequencing machines.
- In 2013, Mats Nilsson's research team published the 'in situ sequencing' method, one of the methods used in the 'Spatial Omics' research method. *
- In 2015, the 'single cell genomic' technology was introduced, a machine that made it possible to measure RNA in individual cells. This made it possible to start classifying different types of cells. Originally, the trick was to make the test tube smaller and smaller until it became as small as the cell. Now there is a machine that easily does the job. You put the cells in a machine that distributes them through small drops of liquid in an oil. Each liquid drop contains a cell and a reagent that makes the RNA molecules identifiable. Each cell also has a label in the form of DNA. The result was a large global project called 'The Human Cell Atlas'.
- In 2020, it became possible to place the cells from the single cell machine in the tissue, using a technique developed in Mats Nilsson's lab, to create a cell map.
* SPATIAL OMICS
Spatial omics is a field of technology, a way of approaching a research question through a combination of methods. You could call it an experimental scientific approach. In 2020, the scientific journal Nature Methods named Spatial transcriptomics the method of the year.
Mats Nilsson's research group
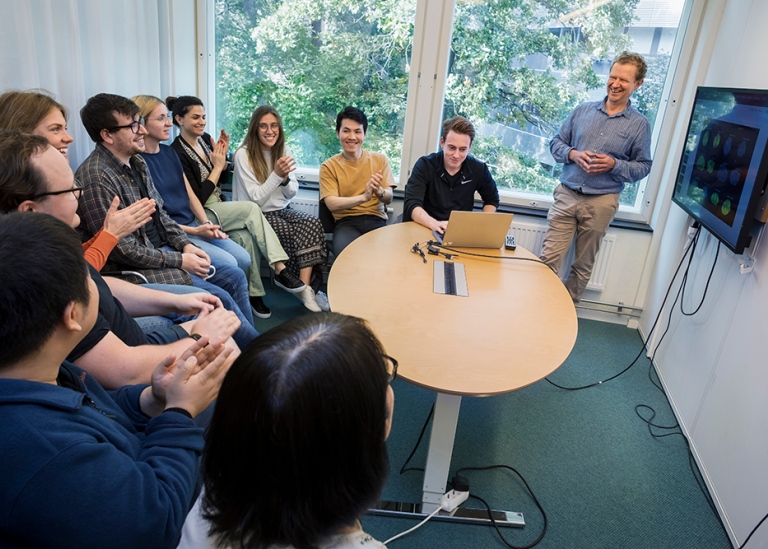
The research team of 12 scientists from around the world - biotechnologists, biomedical scientists, bioinformaticians and a surgeon - is based at Scilifelab. Where the technology needed for such advanced research is. Because of its expertise and access to high-tech equipment, the team also offers its services to groups around the world that have questions and samples, such as medical clinics.
Last updated: February 5, 2024
Source: Translation: Linn Rylander, DBB