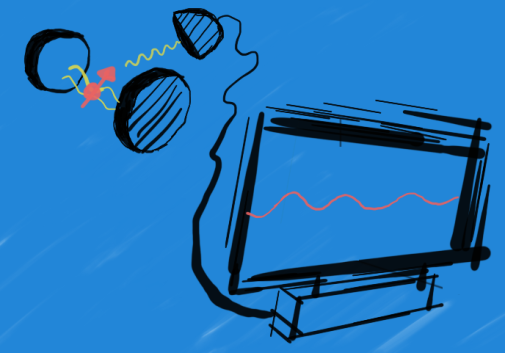
Photon blockade is an exemplary manifestation of the nonlinear light-matter interaction described by the open weakly-driven Jaynes-Cummings (JC) model in its strong coupling limit. In this limit, the spacing between energy levels as defined by the light-matter coupling strength, is much larger than the level widths acquired by the presence of dissipation. While free photons are bosons, in a resonant environment where the input field is tuned to the energy difference of the JC energy levels, multi-photon resonances are created blocking the absorption of any additional photons. This is traditionally what one expects from fermionic statistics. In the current quantum optical configuration modeled by the JC oscillator, the occurring blockade depends on the dynamical balance between input and output, and breaks down by means of a dissipative quantum phase transition [1]. A natural question that comes to mind then is the following: "How is photon blockade organized and monitored?''.
Cristóbal and Themis, in their recent work published by Optics Communications, address this question by assessing the coherence properties of the light scattered off the sides of a driven cavity; the cavity supports a resonant mode which is strongly coupled to the two-level atom. In their work, they find that while a multi-photon resonance is formed by the excitation of a two-level spacing in the oscillator spectrum, the intermediate levels imprint a quantum interference signature which is visible in the intensity correlation function of the scattered field. At the same time, the weak drive induces a further perturbative "dressing'' of the states that have already been "dressed'' by the JC light-matter interaction with its large coupling strength. This so-called "dressing of the dressed states'' produces semiclassical oscillations traceable in the intensity correlation [2]. To visualize the exact steps defining the cascaded process, now, we need to appeal to the first-order coherence and the spectrum of the atomic emission. Since atom-photon superposition states involving up to two photons are routinely within reach in modern cavity and circuit QED experiments (see e.g., [3]), this theoretical study calls for a direct hands-on investigation of the strong-coupling limit and its associated coherence.
[1] H. J. Carmichael, Phys. Rev. X 5, 031028 (2015).
[2] S. S. Shamailov et al., Optics Commun. 283, 766 (2010).
[3] I. Schuster, A. Kubanek, A. Fuhrmanek et al., Nature Phys. 4, 382 (2008).
Direct link to the published article:
https://www.sciencedirect.com/science/article/pii/S0030401821000419